When scientists and engineers use the word materials, they mean any naturally occurring substance manipulated by humans to make things. Beginning with the first metals, discovered by trial and error thousands of years ago, the drive to develop materials that better serve human needs has played a central role in the rise of complex societies.
Modern researchers have moved past haphazard experimentation. Today they examine materials at every level – from the nanoscale to the visible and tangible macroscale – to understand why a material behaves as it does.
At Georgia Tech, investigators unite research capabilities with powerful new tools to develop and characterize novel materials. By pinpointing the complex physical and chemical interactions that control performance, they are creating materials with unique properties.
The White House recently stressed the economic importance of materials expertise when it launched the Materials Genome Initiative, aimed at speeding the pace with which advanced materials move from discovery to industry applications. Georgia Tech is well positioned with the Institute for Materials (IMat), established in 2013 as one of nine interdisciplinary research institutes on campus.
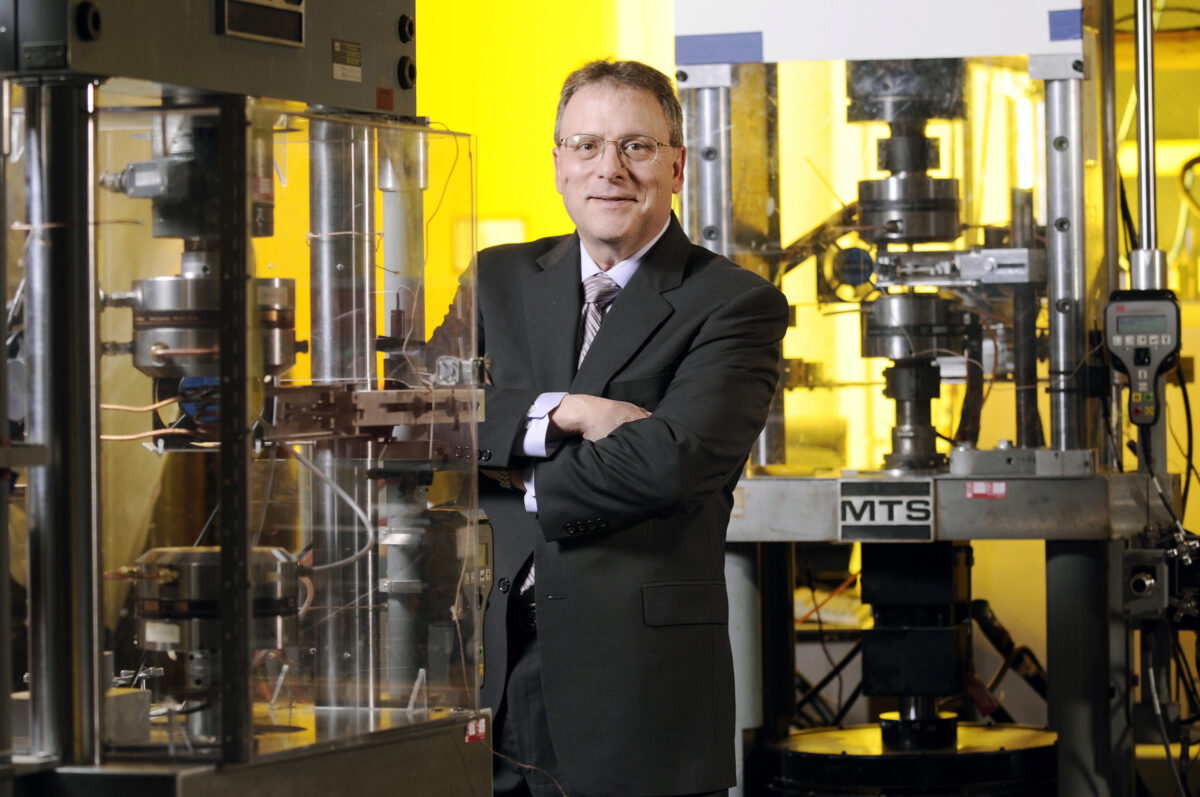
“At Georgia Tech we have some 200 faculty who focus on materials research,” said McDowell, who is the Carter N. Paden Jr. Distinguished Chair in Metals Processing in the Woodruff School of Mechanical Engineering, with a joint appointment in the School of Materials Science and Engineering. ”They tackle a broad range of areas including materials for electronics, infrastructure, energy, environment, transportation, biotechnology, aerospace and defense. The very breadth of that research makes multidisciplinary collaboration both possible and desirable.”
The campus is home to numerous interdisciplinary materials groups – including the Materials Research Science and Engineering Center, the Center for Organic Photonics and Electronics, and the Institute for Electronics and Nanotechnology – that bring together dozens of faculty researchers to focus on core problems.
Materials research at Georgia Tech addresses every type of material, including metals, ceramics, polymers, textiles, composites, nanomaterials, bio-molecular solids – even familiar yet indispensable concrete. And cutting-edge structures that combine very different materials can offer unique capabilities – as in the case of spider silk and graphene oxide, which yield a light, flexible material stronger than steel.
This article presents an overview of materials work from the faculty of the Woodruff School, focusing on a few of the many innovative research projects underway.
Improving Materials for Extreme Conditions
Ensuring Engine Dependability – Everyone wants to be confident that jet engines are completely dependable. Richard W. Neu, a professor in the Woodruff School of Mechanical Engineering, studies the details of exactly this issue.
With funding from the Department of Energy and several multinational corporations, Neu has focused on fatigue and fracture of metallic alloy systems for nearly two decades. He specializes in high temperature fatigue and fracture behavior – how the microstructure of highly stressed metal parts changes over time.
“We’re developing models to capture the evolution of gas turbine engine parts over time, so we can predict how that microstructure will change with operational conditions,” said Neu, who directs the Mechanical Properties Research Laboratory at Georgia Tech.
Neu and his research team are studying gas turbine engines for both aerospace use and for land-based power generation. In both cases, gases in excess of 1,400 degrees Celsius – higher than the melting point of most metals – require active cooling strategies and parts made of special alloys to survive these harsh conditions.
In such demanding environments, the high temperatures and stress always take a toll, Neu said. “Among other investigations, we’ve taken a used engine blade, in service for about three years, and compared its microstructure to an unused blade,” said Neu, who also teaches in the School of Materials Science and Engineering. “And I can tell you, they’re vastly different.”
What’s more, he said, the differences are not uniform. The microstructure of engine parts can vary dramatically depending on the combined temperature and stress cycles – meaning exactly how and when the parts encountered temperature and stress. Neu simulates these complex thermomechanical cycles in the laboratory to characterize the degradation of the material under operational conditions.
The materials that Neu tests are typically nickel-based superalloys, which are widely used in gas turbine engines. More recently, he’s been studying promising new high temperature materials such as gamma titanium aluminides, which are so lightweight that they could be revolutionary for aerospace applications.
Exploiting Microstructure Data – Advanced metal alloys have become indispensable in various emerging technologies – especially where extreme conditions demand new levels of performance and lighter weight. But developing novel alloys is difficult without a thorough understanding of metal microstructure.
“We can no longer afford to depend on the element of luck in developing materials,” said Surya Kalidindi, a professor in the Woodruff School of Mechanical Engineering. “Today, interdisciplinary research has enabled us to capture materials knowledge that makes it much easier for the designer or manufacturing engineer to understand the microstructure – and this knowledge lets them deploy new technology much faster.”
In projects funded by the Department of Defense, Kalidindi and his team are researching ways to improve lightweight structural metals used in the transportation sector. The goal is to increase operating temperatures in service, which would translate to higher efficiency and major fuel savings.
But developing new alloys requires more than familiarity with the relevant metals chemistry, Kalidindi explained. The materials designer needs to understand how the crystals within a metal alloy fit together at the micron level, an interaction that has far-reaching effects on properties.
The solution is a computer database containing in-depth information on the internal makeup of many different materials, he said. The data on these properties are derived from experiments conducted by Kalidindi and many other researchers.
“In some ways you can compare this approach to a fingerprint database, where you can quickly compare a new print coming in to similar ones based on its characteristics,” he said. “We have developed techniques that allow us to represent each microstructure’s characteristics three-dimensionally, so we can look at a new material and see how it is similar to structures on which we already have detailed information.”
In two National Science Foundation-funded projects, Kalidindi is studying development of lighter weight automobile parts made of either high-strength steels or new types of magnesium alloys. Among the challenges is the need to find technologies that can reduce vehicle weight yet cost no more than current techniques.
Materials Reliability in Structures, Infrastructures and Energy – Professor Min Zhou of the Woodruff School of Mechanical Engineering (ME) studies the effects of mechanical, thermal and chemical loading on the behaviors and reliability of structural, infrastructural and functional materials, such as metals, ceramics, semiconductors and composites. One focus involves high strain rate mechanical loading, which can come from several causes, including high-speed machining, impact, penetration, and the explosion of energetic materials.
As part of a federally funded project, Zhou has built a laboratory in the Georgia Tech Manufacturing Institute to investigate how ship structures respond to the effects of underwater explosions. Using a special gas-driven gun, he generates high-pressure waves through water and uses the impulsive loads to analyze the resulting fluid-solid interactions with high-speed digital cameras and laser interferometers.
The goal is to develop new materials for ship construction. Under special consideration are sandwich structures, which are polymer-based composites that are lightweight, inexpensive and highly corrosion resistant.
But such materials must also be highly resistant to heavy weather, encounters with reefs and other threats. Using both experiments and computer simulations, Zhou is designing composite structures that could meet these requirements.
In another project, sponsored by the Army and the Department of Homeland Security, Zhou is working with ME professor David McDowell on infrastructure materials that could offer increased protection against earthquakes, as well as terrorist attacks. The team is using both large-scale experiments and computational modeling techniques to study ultra-high-performance concrete designs that use novel metal fibers for added strength.
Zhou also studies a range of issues related to materials in energy applications. In a project sponsored by the National Research Foundation of Korea, he is addressing problems surrounding the use of silicon to replace graphite in next-generation high-capacity rechargeable lithium ion batteries.
Silicon is a highly desirable replacement for traditional graphite as anodes in lithium-ion batteries, because of its much higher lithium storing capacity. However, it is more prone to mechanical failure through cracking due to large volume changes during charge and discharge. Zhou is developing models that outline approaches for improving the reliability of silicon-based anodes by taking advantage of the size dependence of coupled mechanical chemical diffusional processes in the materials.
Novel Next-Generation Composites
Yet today’s carbon fiber materials have a long way to go before they achieve their full potential, said Satish Kumar, a professor in the School of Materials Science and Engineering. He is leading a four-year, $9.8 million project sponsored by the Defense Advanced Research Projects Agency (DARPA) to improve these materials using nanotechnology in the form of carbon nanotubes.
“It’s likely that carbon fiber materials could be about 10 times stronger than they are presently, so there is tremendous room for further improvement in their tensile and other structural properties,” Kumar said. “By using carbon nanotubes to reinforce carbon fibers, our objective is development of a next-generation carbon fiber with double the tensile strength of today’s strongest carbon fibers.”
In an advanced laboratory established for the current project, Kumar and his team are optimizing techniques for converting polymeric materials into high-strength carbon fiber, using a multi-stage process.
Untreated polymers contain carbon, hydrogen, oxygen and nitrogen, Kumar explained. They can be made into carbon fiber via a selective treatment process called pyrolysis, in which a polymer mix is gradually subjected to both heat and stretching. This treatment eliminates large quantities of hydrogen, oxygen and nitrogen, leaving an increased amount of carbon that makes the fiber stronger.
Kumar modifies this process by adding carbon nanotubes – about one percent by weight – to the polymer mixture before pyrolysis. Among the challenges is finding the best methods for dispersing the carbon nanotube solution uniformly in the polymer mix.
“If the mixing process is fully successful, the carbon nanotubes will reorient the crystals within the polymer in a uniform direction,” he said. “The altered molecular structure has the potential to make the resulting carbon fiber much stiffer and stronger.”
Understanding Structures to Aid Materials Development
Such films can be used in applications that include optical coatings, batteries, solar cells, semiconductors and micro-electromechanical systems (MEMS). In addition, thin films can be used to protect other materials against degradation such as corrosion or harmful reactions with the environment.
One of Graham’s current research goals involves developing defect-free coatings using a method called atomic layer deposition (ALD), which deposits the films in a layer by layer fashion and gives unique control over film thickness and film composition. He is also researching layers that can be used to allow uniform growth of these films using ALD on virtually any material, including plastics, metals and organic electronics.
In work sponsored by the Department of Energy, Graham and his team are collaborating with professor Bernard Kippelen of the School of Electrical and Computer Engineering in the use of ALD to create barrier films that can protect flexible and organic electronics from being degraded by water vapor and oxygen in the ambient environment. They have found that materials including aluminum oxide and titanium oxide perform well in protecting the electronics underneath.
Testing these oxides includes measuring water vapor transport rates through very thin layers to gauge protection levels. Graham and his team are also looking at the use of these oxides in flexible electronics, testing how far these thin films can be bent or stretched before they develop cracks.
“One of the things we have found is that the thicker a film is, the less strain it takes to crack it, so the ultra-thin films are better,” Graham said. “That finding could benefit industry, because putting down a 20-nanometer thick film takes less time and material than producing a 50-nanometer thick film.”
Among other projects, Graham is researching the use of oxide films in the development of novel electrodes for organic electronics. The result could be more efficient and stable electrodes for organic solar cells, which is an emerging technology for future photovoltaic systems.
Developing Metal Foams – Antonia Antoniou, an assistant professor in the Woodruff School of Mechanical Engineering, studies the mechanics of materials at the nanoscale. She and her research team are synthesizing and studying metal foams, which are materials with nano-sized pores that behave like tiny sponges. The aim is to assess the unusual mechanical properties of these nanopore formations. Their intricate three-dimensional structure contains a large amount of surface area, along with a granular composition that offers myriad interfaces.
Such foams hold promise for applications including battery electrodes or supercapacitors, catalysts that increase chemical reactions, and tiny sensors.
Antoniou’s techniques enable structures to self-assemble at the nanoscale. She and her team start with a mixture of two or more metal elements, and then selectively use a corrosive environment to dissolve one or more of them. The result is an intricate porous network with special properties.
The researchers test the foams’ mechanical properties using a tiny probe called a nanoindenter. They also use electron microscopes to image the surface and view changes taking place at the nanoscale.
“The rules tend to change when you reach the atomic level,” Antoniou said. “Unlike a bulk alloy, these foams often have gigapascal strength, which is a very high level of strength for a metal and could enable certain challenging applications.”
Antoniou works with a variety of metals, especially platinum, copper and molybdenum. Collaborating with scientists at Georgia Tech and several other universities, she is investigating applying these foams to the needs of several industries.
In one National Science Foundation-sponsored project, the group is studying applications that could be useful to the nuclear industry. The foams’ innate strength lets them tolerate a significant amount of radiation, suggesting potential applications such as protective coatings.
“It’s important to remember that successful applications are based on understanding these materials at a fundamental level,” she said. “By synthesizing them, we exercise control over the structure, and then by testing them, we can discover unique behaviors.”
“We call ferroelectrics smart materials, because they react to many different external fields – not only mechanical but also electrical and thermal – and they lend themselves to many applications including sensors, actuators and energy harvesting,” said Bassiri-Gharb, who has a joint appointment in the School of Materials Science and Engineering. “My group is specifically trying to understand the fundamental behavior of ferroelectric materials at the very small scale.”
Bassiri-Gharb and her research team are pursuing multiple research projects related to ferroelectrics. They’re working extensively on electromechanical response in piezoelectric materials, which produce a charge when mechanically stimulated. In a Small Business Innovation Research (SBIR) project funded by the Air Force, Bassiri-Gharb is working with a company to develop large-scale production of ferroelectric nanotubes to harvest energy from human stepping motion. The challenge is to distribute the material in a network of piezoelectric islands rather than in one piece. That configuration creates pathways that allow the maximized amount of the generated electric charge to flow to collection points such as batteries or capacitors.
In a collaborative project with Oak Ridge National Laboratory, Bassiri-Gharb and her team are working on understanding multiscale coupling of mechanical, electrical and chemical properties in oxide thin films – specifically as it applies to miniaturization of energy technologies including multilayer capacitors, batteries, and fuel cell devices.
“We’ve learned a great deal from this work, including the interaction between the piezoelectric thin film and its inactive silicon substrate” she said. “Our research shows that as we drastically reduce the thickness of the silicon, the piezoelectric response gets much larger.”
Georgia Tech Institute for Materials
The Georgia Tech Institute for Materials (IMat) was launched with one core mission: to foster materials-related research throughout the campus. Its June 2013 announcement came exactly two years after the White House launched its Materials Genome Initiative for Global Competitiveness.
The Materials Genome aids U.S. economic development by providing training and infrastructure to help U.S. innovators discover, develop and deploy advanced materials more quickly. The Georgia Tech move supports President Barack Obama’s call for faster movement of advanced materials from laboratory to application.
“Traditionally, it’s taken about 15 years to get a new materials discovery into an advanced product, but it only takes 18 to 36 months to design that new product on computers,” said David McDowell, IMat’s executive director and a Regents’ Professor in the Woodruff School of Mechanical Engineering. “There’s a big disconnect there, and we need to integrate materials design and development much more tightly with new product development.”
IMat is focusing on collaborative, interdisciplinary linkages to achieve new levels of cooperation. Its job involves linking materials-related research within Georgia Tech’s academic units and the Georgia Tech Research Institute (GTRI) to industry, government and academic research laboratories across the nation.
Through this collaborative network, IMat connects the expertise of investigative teams at Georgia Tech with the materials community outside, to help move research advances forward more rapidly. At the same time, it seeks to build bridges between Georgia Tech materials research and important application areas such as energy, manufacturing, nanotechnology, bioengineering and the biosciences.
IMat is one of nine Interdisciplinary Research Institutes (IRIs) under the leadership of Georgia Tech’s Executive Vice President for Research, Stephen E. Cross. Each IRI spans Georgia Tech units to bring together researchers working in a core area. In addition, the IRIs help government and industry navigate Georgia Tech’s myriad activities and connect with researchers, students and laboratory capabilities.
“The benefits of materials-based advances over the last 20 years are now a part of our everyday lives – lifesaving medical technologies, the computers and phones we can’t live without, our more efficient and safer vehicles, and much more,” said McDowell. “Materials research both discovers new materials and uses existing materials in new and enhanced ways. Our continued growth in a competitive global economy depends on performing effectively in these research areas and then applying those insights to real-world applications.”
Research projects mentioned in this article are supported by sponsors that include the National Science Foundation (NSF), Office of Naval Research (ONR), Department of Energy (DOE), Department of Homeland Security (DHS), U.S. Air Force (USAF), Air Force Office of Scientific Research (AFOSR), U.S. Army (USA), Army Research Office (ARO), Oak Ridge National Laboratory (ORNL), Defense Threat Reduction Agency (DTRA) and the Defense Advanced Research Projects Agency (DARPA). Any opinions, findings, conclusions or recommendations expressed in this publication are those of the principal investigators and do not necessarily reflect the views of the NSF, ONR, DOE, DHS, USAF, AFOSR, USA, ARO, ORNL, DTRA or DARPA.
Georgia Tech Research News
Writer: Rick Robinson
Photography: Gary Meek
Read full article here for stories on other Georgia Tech faculty members outside the Woodruff School